Now you understand how scuffing along a carpet in socks builds up electrons on the body, and how this negative electric charge affects other things (like your cat) when you reach a finger out to touch them. You also know how opposite charges attract and like charges repel, and the difference between balanced charges and unbalanced charges.
We’re going to dive into studying force fields. You may wonder what force fields have to do with a serious examination of physics like the one in this lesson. You probably consider force fields to be something you might hear about in a science fiction scene such as…
Please login or register to read the rest of this content.
Overall, Maxwell’s four equations describe the fundamentals of electricity and magnetism. However, one look at these mathematical equations can make a high school student run screaming from the room, so we’re not going to dive into the sophisticated mathematics of the equations themselves, but rather what they really tell us about the relationships between the electric and magnetic fields.
Please login or register to read the rest of this content.
A field changes the nature of the space surround the thing producing the field. A magnet produces a magnetic field which changes the nature of the space around it so that other magnets and magnetic objects are now influenced by it. Some magnetic fields (and other fields) are stronger than others, and now we’re going to learn how to measure the field strength of electric fields.
The electric field is a vector (it has magnitude and direction), and this is how you do it:
Please login or register to read the rest of this content.
Michael Faraday was the first to come up with the idea about electric fields. He thought of the space around a charged object as being filled with lines of force. He was trying to figure out a pattern that represented what the electric field looked like by imagining the electric field as a bubble around a charged object and how it would interact with another object that enters into that bubble. This is a little different than imagining a charge interacting with a charge. There’s a field interaction between the two charges. Every charge creates a bubble around it that in turn, affects the space within that bubble.
Please login or register to read the rest of this content.
Have you wrapped your mind around static electricity yet? You should understand by now how scuffing along a carpet in socks builds up electrons, which eventually jump off in a flurry known as a spark. And you also probably know a bit about magnets and how magnets have north and south poles AND a magnetic field (more on this later). Did you also know that electrical charges have an electrical field, just like magnets do?
It’s easy to visualize a magnetic field, because you’ve seen the iron filings line up from pole to pole. But did you know that you can do a similar experiment with electric fields?
Here’s what you need:
- dried dill (spice)
- vegetable or mineral oil
- 2 alligator wires
- static electricity source (watch video first!)
Click here to go to next lesson on Weird Shapes and Field Lines.
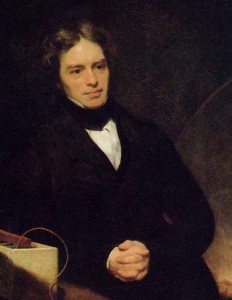
Michael Faraday also discovered how you can have an electric field inside a charged conductor. Image you have a room within a room, and the inner room is made completely of metal. You can sit in the inner room with a static charge detector (like an electroscope), and when you charge the surfaces of both rooms, you’ll see sparks flying between the two rooms, but it’s peacefully (electrically speaking) quiet in the inner room. No charge is detected inside the inner room with your electroscope. You can have a bolt of lightning strike the inner room, but it still doesn’t register a charge inside the inner room. Why is that?
The inner room I’ve just described is called a “Faraday Cage”, and it’s often seen at science and magic shows because it absolutely defies common sense, until you really think about it. The inner room is shielding you from electric fields. Any closed conducting surface can be a Faraday cage. By closed, I mean electrically speaking. The cage can be a cage made of bars or chicken wire, but it’s still got to be electrically closed. During the experiment, you can even run your hands on the inside of the room and still not get a shock from the sparks flying around between the rooms!
Please login or register to read the rest of this content.
You’re already familiar with two different kinds of potential energy: elastic (like the energy stored in a rubber band) and gravitational (the energy stored in height). Now let’s take a look at electrical potential energy stored in an electric field:
Please login or register to read the rest of this content.
If you have a Fun Fly Stick, then pull it out and watch the video below. If not, don’t worry – you can do most of these experiments with a charged balloon (one that you’ve rubbed on your hair). Let’ play with a more static electricity experiments, including making things move, roll, spin, chime, light up, wiggle and more using static electricity!
Please login or register to read the rest of this content.
Click here to go to next lesson on Alien Detector.
This simple FET circuit is really an electronic version of the electroscope. This “Alien Detector” is a super-sensitive static charge detector made from a few electronics parts. I originally made a few of these and placed them in soap boxes and nailed the lids shut and asked kids how they worked. (I did place a on/off switch poking through the box along with the LED so they would have ‘some’ control over the experiment.)
This detector is so sensitive that you can go around your house and find pockets of static charge… even from your own footprints! This is an advanced project for advanced students.
You will need to get:
- 9V battery clip (and a 9V battery)
- MPF 102 (buy 2 – one for back up)
- LED (any regular LED works fine)
Click here to go to next lesson on Lightning.
What causes lightning, and how can we protect ourselves from strikes? In many textbooks, you’ll read about how clouds become electrically charged through friction in the moist air, but the truth is, scientists still don’t fully understand how and why lightning happens the way it does. But here’s what we do know: lightning happens when the positive and negative charges in a cloud become polarized. That is, the (extra) positive charges move to the top of the cloud and the (extra) negative charges move to the bottom of the cloud, usually by friction of the water vapor molecules in the cloud.
As the water molecules rise, electrons are stripped off and add to the charge of the cloud. The cloud can become ever more polarized if the rising water vapor freezes. The frozen particles clump together and take on a negative charge inside, positive charge on the outside, which rips the clumps apart to further polarize the cloud. The more polarized the cloud is, the more its electric field affects the space around it. The electrons on the surface of the Earth underneath the cloud are repelled by the bottom of the cloud, which creates a positive charge on the surface under the cloud. Trees. houses, cars, and people take on a positive charge as the cloud passes by. Now we’re set up for a lightning strike.
Please login or register to read the rest of this content.
The way charges attract or repel each other can be described as a force. A charge can exert a push or pull on another charge depending on if the charges are positive or negative. How much force they exert can be figured out using Coulomb’s Law of Electric Force, which is:
where C = 8.99 x 109 Nm2/C2
Please login or register to read the rest of this content.
You can’t do an experiment on the planet without gravity playing some part of it (albeit sometimes so small you can ignore gravitational effects) since we’re in the Earth’s gravitational field. The electrical forces will add another force vector to our FBD that can be used when we look at how objects move in reaction to the forces.
Let’s take a look at how this works:
Please login or register to read the rest of this content.
Wasn’t that incredible how much force was present just between the two? After I figured that out, I wanted to know how much “push” was present between two protons in the nucleus. If you think about it, there’s really no reason for the protons to stick together inside the nucleus because they are all positively charged. Let’s take a look at the iron atom as we figure this out…
Please login or register to read the rest of this content.
Click here to go to next lesson on Triboelectric series.
The Triboelectric Effect is a type of electrification that happens when you rub two different materials together and then separate them. Often, one will take on a positive charge and the other a negative. But how do you know which is going to be which?
Please login or register to read the rest of this content.
Click here to go to next lesson on Electrostatic Motors.
You can use the idea that like charges repel (like two electrons) and opposites attract to move stuff around, stick to walls, float, spin, and roll. Make sure you do this experiment first.
I’ve got two different videos that use positive and negative charges to make things rotate, the first of which is more of a demonstration (unless you happen to have a 50,000 Volt electrostatic generator on hand), and the second is a homemade version on a smaller scale.
Did you know that you can make a motor turn using static electricity? Here’s how:
Please login or register to read the rest of this content.
Click here to go to next lesson on Coulomb’s Law.
Induction is another way to create a charge in an object. You can charge an object by induction without even touching it. I’ve got a couple of really neat experiments that will show you how this works, but here’s the basic idea: when you have two metal objects, like two soda cans, standing upright on a foam slab and just touching each other (so they are insulated from the table but in contact with each other), you can bring a charged balloon close to one of them and see a really interesting effect: the can closest to the charged balloon (which has a negative charge) will take on a positive charge, and the soda can furthest from the balloon will take on a negative charge. And when you separate the two cans, the charges on each will be evenly distributed over the surface of each can and remain polarized (the further can keeps its negative charge and the closer can keeps its positive charge). That’s charging by induction!
Please login or register to read the rest of this content.
When high energy radiation strikes the Earth from space, it’s called cosmic rays. To be accurate, a cosmic ray is not like a ray of sunshine, but rather is a super-fast particle slinging through space. Think of throwing a grain of sand at a 100 mph… and that’s what we call a ‘cosmic ray’. Build your own electroscope with this video!
Please login or register to read the rest of this content.
Jupiter not only has the biggest lightning bolts we’ve ever detected, it also shocks its moons with a charge of 3 million amps every time they pass through certain hotspots. Some of these bolts are cause by the friction of fast-moving clouds. Today you get to make your own sparks and simulate Jupiter’s turbulent storms.
Electrons are too small for us to see with our eyes, but there are other ways to detect something’s going on. The proton has a positive charge, and the electron has a negative charge. Like charges repel and opposite charges attract.
Materials
- Foam plate
- Foam cup
- Wool cloth or sweater
- Plastic baggie
- Aluminum pie pan
- Aluminum foil
- Film canister or M&M container
- Nail (needs to be a little longer than the film canister)
- Hot glue gun or tape
- Water
Click here to go to next lesson on Easy Photoelectric Effect Experiment
Einstein received a Nobel Prize for figuring out what happens when you shine blue light on a sheet of metal. When he aimed a blue light on a metal plate, electrons shot off the surface. (Metals have electrons which are free to move around, which is why metals are electrically conductive. More on this in Unit 10).
When Einstein aimed a red light at the metal sheet, nothing happened. Even when he cranked the intensity (brightness) of the red light, still nothing happened. So it was the energy of the light (wavelength), not the number of photons (intensity) that made the electrons eject from the plate. This is called the ‘photoelectric effect’. Can you imagine what happens if we aim a UV light (which has even more energy than blue light) at the plate?
This photoelectric effect is used by all sorts of things today, including solar cells, electronic components, older types of television screens, video camera detectors, and night-vision goggles.
This photoelectric effect also causes the outer shell of orbiting spacecraft to develop an electric charge, which can wreck havoc on its internal computer systems.
A surprising find was back in the 1960s, when scientists discovered that moon dust levitated through the photoelectric effect. Sunlight hit the lunar dust, which became (slightly) electrically charged, and the dust would then lift up off the surface in thin, thread-like fountains of particles up ¾ of a mile high.
Please login or register to read the rest of this content.
Click here to go to next lesson on Charging by Induction and Conduction.
Ygou can also charge objects by conduction. You’ve actually already done with without really thinking about it. The foil on the wire coat hanger in the electroscope was being charged by conduction. When you touch a charged balloon to the foil ball on the electroscope, that’s a charge by conduction. If you were to get the charged balloon really close but not touching the foil ball, that would be charging by induction. (See the difference?) Charging by conduction just means that you need to touch the electrically neutral object to the one that is charged to transfer the charge. It’s charge by contact.
With charge by induction, it’s the forces due to likes repelling and opposites attracting that cause the charge in objects. With conduction, it’s the actual movement of electrons to the object that make the charge in the object. This is obvious if you think about touching two soda cans together, since they are both made out of a material that allows electrons to move about freely within the material (on the surface of the object). But what about two insulators, like two foam plates? What happens then?
Please login or register to read the rest of this content.
This experiment is just for advanced students. If you guessed that this has to do with electricity and chemistry, you’re right! But you might wonder how they work together. Back in 1800, William Nicholson and Johann Ritter were the first ones to split water into hydrogen and oxygen using electrolysis. (Soon afterward, Ritter went on to figure out electroplating.) They added energy in the form of an electric current into a cup of water and captured the bubbles forming into two separate cups, one for hydrogen and other for oxygen.
This experiment is not an easy one, so feel free to skip it if you need to. You don’t need to do this to get the concepts of this lesson but it’s such a neat and classical experiment (my students love it) so you can give it a try if you want to. The reason I like this is because what you are really doing in this experiment is ripping molecules apart and then later crashing them back together.
Have fun and please follow the directions carefully. This could be dangerous if you’re not careful. The image shown here is using graphite from two pencils sharpened on both ends, but the instructions below use wire. Feel free to try both to see which types of electrodes provide the best results.
Please login or register to read the rest of this content.
Click here to go to next lesson on Molecules and Atoms.
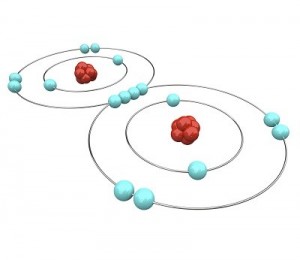
Let’s try another way to look at this. You’re playing miniature golf and you come to the old wind mill hole. Your friend takes a shot and since the blades of the windmill are going nice and slow he gets the ball right through. Now it’s your turn. Suddenly you hear a zap and a pow and sparks go flying. Something has gone wrong with the wind mill and it starts spinning at amazing speeds. You decide to give it a try and hit the ball towards the wind mill.
Well since it is spinning out of control, those blades now form almost a solid disk so that there is no way your ball can get through the wind mill. Electrons do the same thing. They move so fast that even though there may not be many of them, they form a shell that can’t be penetrated. (To be clear, particles that are smaller than an atom can go through the shells and pop out the other side.)
Let’s go a little further with this shell thing. An atom can have as few as one and as many as seven shells. Imagine our balloon again. Now there can be a balloon inside of a balloon inside a balloon and so on. Up to seven balloons! Each balloon, whoops, I mean shell, can have only so many electrons in it. This simple equation 2n2 tells you how many electrons can be in each shell. The n stands for the number of the shell.
The first shell can have up to 2 x 1(first shell)2 or 2 electrons. The second shell can have up to 2 x 2(second shell)2 or 8 electrons. The third shell can have up to 2 x 32 or 18 electrons. The fourth shell can have up to 2 x 42 or 32 electrons. All the way up to the seventh shell which can have 2 x 72 or 98 electrons!
One last thing about shells, the shells have to be full before the electrons will go to the next shell. A helium atom will have two electrons. Both of them will be in the first shell. A Lithium atom will have three electrons. Two will be in the first shell and one (since the first shell is filled) will be in the second shell.
Electrons provide the size and stability of the atom and, as such, the mass and the structure of all matter. Electrons are also the key to all electromagnetic energy. But wait, that’s not all! It is the number of electrons in an atom that determines if and how atoms come together to form molecules. Electrons determine how and what matter will be.
Atoms like to feel satisfied and they feel satisfied if they are “full”. An atom is “full” if its outer electron shell has as many electrons as it can hold or if there are eight or a multiple of eight (16, 24 etc.) electrons in the outer shell. This is called the octet rule and works most of the time, but is not perfect.
If an atom is not full, it is not satisfied. An unsatisfied atom needs to do something with its electrons to be happy. Luckily atoms are very friendly and love to share. Most atoms are not satisfied as individuals. The oxygen atom has six electrons in its outer shell. It needs eight electrons to be satisfied.
Luckily, two Hydrogen atoms happen by. Each one of them has only one electron in its outer shell and needs one more to be satisfied. If both Hydrogens share their one electron with the Oxygen, the oxygen has eight electrons and is satisfied. Also, if the Oxygen shares an electron with each Hydrogen, then both Hydrogens are satisfied as well. Just like your mother told you, it’s nice to share. It is this sharing of electrons that makes atoms come together to form molecules.
Click here to go to next lesson on Electrostatic Charge.
[/am4show]
To summarize, protons and neutrons are in the nucleus of an atom, and tightly bound together. The proton has a positive charge while the neutron has no charge, and both of them are much larger than the electron. The tiny electron is outside the nucleus and weakly bound to the atom and carries a negative a charge.
Please login or register to read the rest of this content.
Let’s go back to rubbing a balloon on your head. When you do this and bring it close to objects like a thin stream of water trickling out of the faucet, or small its of paper, or bubbles in the air, or even a ping pong ball on the table, did you notice now you can influence things? You can make water flick and spray, paper jump up and down, and bubbles and ping pong balls will follow your every move. But why is that?
Please login or register to read the rest of this content.
An electrical circuit is like a NASCAR raceway. The electrons (racecars) zip around the race loop (wire circuit) superfast to make stuff happen. Although you can’t see the electrons zipping around the circuit, you can see the effects: lighting up LEDs, sounding buzzers, clicking relays, etc.
There are many different electrical components that make the electrons react in different ways, such as resistors (limit current), capacitors (collect a charge), transistors (gate for electrons), relays (electricity itself activates a switch), diodes (one-way street for electrons), solenoids (electrical magnet), switches (stoplight for electrons), and more. We’re going to use a combination diode-light-bulb (LED), buzzers, and motors in our circuits right now.
A CIRCUIT looks like a CIRCLE. When you connect the batteries to the LED with wire and make a circle, the LED lights up. If you break open the circle, electricity (current) doesn’t flow and the LED turns dark.
LED stands for “Light Emitting Diode”. Diodes are one-way streets for electricity – they allow electrons to flow one way but not the other.
Remember when you scuffed along the carpet? You gathered up an electric charge in your body. That charge was static until you zapped someone else. The movement of electric charge is called electric current, and is measured in amperes (A). When electric current passes through a material, it does it by electrical conduction. There are different kinds of conduction, such as metallic conduction, where electrons flow through a conductor (like metal) and electrolysis, where charged atoms (called ions) flow through liquids.
Please login or register to read the rest of this content.
Click here to go to next lesson on Why does metal conduct electricity?
Make yourself a grab bag of fun things to test: copper pieces (nails or pipe pieces), zinc washers, pipe cleaners, Mylar, aluminum foil, pennies, nickels, keys, film canisters, paper clips, load stones (magnetic rock), other rocks, and just about anything else in the back of your desk drawer.
Certain materials conduct electricity better than others. Silver, for example, is one of the best electrical conductors on the planet, followed closely by copper and gold. Most scientists use gold contacts because, unlike silver and copper, gold does not tarnish (oxidize) as easily. Gold is a soft metal and wears away much more easily than others, but since most circuits are built for the short term (less than 50 years of use), the loss of material is unnoticeable.
Please login or register to read the rest of this content.
Click here to go to next lesson on Liquid Conductors.
When an atom (like hydrogen) or molecule (like water) loses an electron (negative charge), it becomes an ion and takes on a positive charge. When an atom (or molecule) gains an electron, it becomes a negative ion. An electrolyte is any substance (like salt) that becomes a conductor of electricity when dissolved in a solvent (like water).
This type of conductor is called an ‘ionic conductor’ because once the salt is in the water, it helps along the flow of electrons from one clip lead terminal to the other so that there is a continuous flow of electricity.
This experiment is an extension of the Conductivity Tester experiment, only in this case we’re using water as a holder for different substances, like sugar and salt. You can use orange juice, lemon juice, vinegar, baking powder, baking soda, spices, cornstarch, flour, oil, soap, shampoo, and anything else you have around. Don’t forget to test out plain water for your ‘control’ in the experiment!
Please login or register to read the rest of this content.
Click here to go to next lesson on Superconductors.
When I was in 10th grade, my teammate and I designed what we thought was pretty clever: a superconductor roller coaster, which we imagined would float effortlessly above its magnetic track. Of course, our roller coaster was only designed on paper, because yttrium barium copper oxide ceramics had only just been discovered by top scientists.
Did you notice how it was smoking in the video? That’s because it was so cold! The usual problem with superconductors is that they need to be incredibly cold in order to exhibit superconductive properties. Yttrium barium copper oxide (YBa2Cu3O7) was the first compound that used liquid nitrogen for cooling, making superconductors a lot less expensive to work with – you no longer needed a cryogenic lab in order to levitate objects above a magnet.
Recently, scientists have found a way to make an amazing superconductor by taking a single crystal sapphire wafer and coating it with a thin (~1µm thick) ceramic material (yttrium barium copper oxide). Normally, the ceramic layer has no interesting magnetic or electrical properties, but that’s when you’re looking at it at room temperature. If you cool this material below -185ºC (-301ºF), it turns out that the ceramic material becomes a superconductor, meaning that it conducts electricity without resistance, with no energy loss. Zero. That’s what makes it a ‘superconductor’.
To further understand superconductivity, it’s helpful to understand what normally happens to electricity as it flows through a wire. As you may know, energy cannot be created or destroyed, but can be changed from one form to another.
In the case of wires, some of the electrical energy is changed to heat energy. If you’ve ever touched a wire that had been in use for a while, and discovered it was hot, you’ve experienced this. The heat energy is a waste. It simply means that less electricity gets to its final destination.
This is why superconductivity is so cool (no pun intended.) By cooling things down to temperatures near absolute zero, which is as low as temperatures can get, you can create a phenomenon where electricity flows without having any of it converted to heat.
Why do superconductors float above magnets?
Scientists also figured out that superconductors and magnetic field really do not like each other. The Meissner effect happens when a superconductor expels all its magnetic fields from inside.
However, if you make your superconductor thin enough, you can get the magnetic field to penetrate in discrete quantities (this is real quantum physics now) called flux tubes (the blue lines that go through the disc).
Inside each of the magnetic flux tubes, the superconductivity is destroyed, but the superconductor tries to keep the magnetic tubes pinned in weak areas and any movement of the superconductor itself (like if you pushed it) causes the flux tubes to move, and this is what traps (or locks) the superconductor in midair.
If you’d like to experiment with superconductors yourself, check out this information.
Click here to go to next lesson on The difference between polarizing and charging.
Have you ever had a bad hair day? Did you happen to notice if the air was drier or wetter weather on those days? Usually folks have bad hair days when the air is drier, which is when static charge can build up more easily. Some folks notice every time they touch a doorknob, slide down a plastic slide, or scuff along the carpet in socks that they get zapped. Since there’s less water vapor in the air on drier days, there’s more of a chance for static charge to build up. The water molecule dissipates the static charge, and the more wet the air is (humid), the less static build up there is. Static electricity experiments are really hard to do on humid days, especially if it’s raining outside!
Please login or register to read the rest of this content.
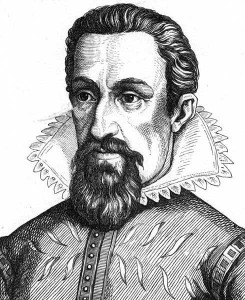
Johannes Kepler, a German mathematician and astronomer in the 1600s, was one of the key players of his time in astronomy. Among his best discoveries was the development of three laws of planetary orbits. He worked for Tycho Brahe, who had logged huge volumes of astronomical data, which was later passed onto to Kepler. Kepler took this information to design and develop his ideas about the movements of the planets around the Sun.
Kepler’s 1st Law states that planetary orbits about the Sun are not circles, but rather ellipses. The Sun lies at one of the foci of the ellipse.
Well, almost.
Newton’s Laws of Motion state that the Sun can’t be stationary, because the Sun is pulling on the planet just as hard as the planet is pulling on the Sun. They are yanking on each other. The planet will move more due to this pulling because it is less massive. The real trick to understanding this law is that both objects orbit around a common point that is the center of mass for both objects. If you’ve ever swung a heavy bag of oranges around in a circle, you know that you have to lean back a bit to balance yourself as you swing around and around. It’s the same principle, just on a smaller scale.
Please login or register to read the rest of this content.
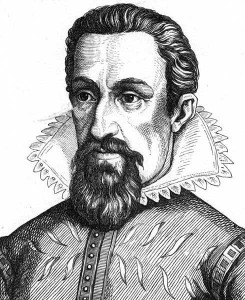
Kepler’s Laws of planetary orbits explain why the planets move at the speeds they do. You’ll be making a scale model of the solar system and tracking orbital speeds.
Kepler’s 1st Law states that planetary orbits about the Sun are not circles, but rather ellipses. The Sun lies at one of the foci of the ellipse. Kepler’s 2nd Law states that a line connecting the Sun and an orbiting planet will sweep out equal areas in for a given amount of time. Translation: the further away a planet is from the Sun, the slower it goes.
Please login or register to read the rest of this content.
If one of Kepler’s Laws describe the orbits of satellites as being an elliptical orbit, you might be wondering what an ellipse is! Here’s a really neat way to make an ellipse using a pencil and a rubber band:
Click here to go to next lesson on Applying Kepler’s Laws.
[/am4show]
An important concept to understand is that a satellite is a projectile, meaning that only the force of gravity is acted on it (once it’s launched). In order to maintain it’s orbit, a satellite needs to fall continuously at the same rate that the earth is curving away from it.
Please login or register to read the rest of this content.
The Hubble Space Telescope (HST) zooms around the Earth once every 90 minutes (about 5 miles per second), and in August 2008, Hubble completed 100,000 orbits! Although the HST was not the first space telescope, is the one of the largest and most publicized scientific instrument around. Hubble is a collaboration project between NASA and the ESA (European Space Agency), and is one of NASA’s “Great Observatories” (others include Compton Gamma Ray Observatory, Chandra X-Ray Observatory, and Spitzer Space Telescope). Anyone can apply for time on the telescope (you do not need to be affiliated with any academic institution or company), but it’s a tight squeeze to get on the schedule.
Hubble’s orbit zooms high in the upper atmosphere to steer clear of the obscuring haze of molecules in the sea of air. Hubble’s orbit slowly decays over time and begins to spiral back into Earth until the astronauts bump it back up into a higher orbit.
But how does a satellite stay in orbit? Try this experiment now:
Materials:
- marble
- paper
- tape
A satellite is an object that does around a planet, a star, or other similar object. Here’s how you can figure out the net force of a satellite as well as the velocity of the satellite, since the only force applied to the satellite is from gravity:
Please login or register to read the rest of this content.
Callisto is one of Jupiter’s moons. Would it be really cool to be able to approximate the size of Jupiter based on watching the motion of Callisto? For example, if you knew how long it took Callisto to orbit around Jupiter, and the furthest distance it traveled away from it (both of which you could measure from a backyard telescope)? Here’s how:
Please login or register to read the rest of this content.
So, here’s a question. If you are “weightless” in space, do you still have mass? Yes, the amount of stuff you’re made of is the same on Earth as it is in your space ship. Mass does not change but since weight is a measure for how much gravity is pulling on you, weight will change. Did you notice that I put weightless in quotation marks? Wonder why?
Please login or register to read the rest of this content.
A binary system exists when objects approach each other in size (and gravitational fields), the common point they rotate around (called the center of mass) lies outside both objects and they orbit around each other. Astronomers have found binary planets, binary stars, and even binary black holes.
The path of a planet around the Sun is due to the gravitational attraction between the Sun and the planet. This is true for the path of the Moon around the Earth, and Titan around Saturn, and the rest of the planets that have an orbiting moon.
Materials
- Soup cans or plastic containers with holes punched (like plastic yogurt containers, butter tubs, etc.)
- String
- Water
- Sand
- Rocks
- Pebbles
- Baking soda
- Vinegar
Today you get to learn how to read an astronomical chart to find out when the Sun sets, when twilight ends, which planets are visible, when the next full moon occurs, and much more. This is an excellent way to impress your friends.
The patterns of stars and planets stay the same, although they appear to move across the sky nightly, and different stars and planets can be seen in different seasons.
Materials:
- Printout of Stargazer’s Almanac
- Pencil
- Tape and scissors (optional)
- Ruler
What would happen if our solar system had three suns? Or the Earth had two moons? You can find out all these and more with this lesson on orbital mechanics. Instead of waiting until you hit college, we thought we'd throw some university-level physics at you... without the hard math.
Please login or register to read the rest of this content.
If you watch the moon, you’d notice that it rises in the east and sets in the west. This direction is called ‘prograde motion’. The stars, sun, and moon all follow the same prograde motion, meaning that they all move across the sky in the same direction.
However, at certain times of the orbit, certain planets move in ‘retrograde motion’, the opposite way. Mars, Venus, and Mercury all have retrograde motion that have been recorded for as long as we’ve had something to write with. While most of the time, they spend their time in the ‘prograde’ direction, you’ll find that sometimes they stop, go backwards, stop, then go forward again, all over the course of several days to weeks.
Here are videos I created that show you what this would look like if you tracked their position in the sky each night for an year or two.
Please login or register to read the rest of this content.
Click here to go to next lesson on Potential Energy in the Stars.
In our physics problems so far, we’ve kept the objects close to the earth so that the acceleration due to gravity g remains constant, and we defined the potential energy of an object on the surface of the earth to be zero. What if we look up and see three stars in a system and want to find out the gravitational potential energy of the system?
Please login or register to read the rest of this content.
Here’s how you put it all together and figure out the total energy of the system. This is useful when you’re trying to figure out something that you can’t otherwise solve for… let me show you with a set of videos here. Remember, for satellites the only force we have on the object is due to gravity, so the external work force term always goes to zero like this:
Please login or register to read the rest of this content.
Einstein once said: “I was sitting in a chair in the patent office at Bern when all of the sudden a thought occurred to me: If a person falls freely, he will not feel his own weight. I was startled. This simple thought made a deep impression on me. It impelled me toward a theory of gravitation.”
This led Einstein to develop his general theory of relativity, which interprets gravity not as a force but as the curvature of space and time. This topic is out of the scope for our lesson here, but you can explore more about it in this lesson.
The fundamental principle for relativity is the principle of equivalence, which says that if you were locked up in a box, you wouldn’t tell the difference between being in a gravitational field and accelerating (with an acceleration value equal to g) in a rocket.
The same thing is also true if you were either locked in a box, floating in outer space or in an elevator shaft experiencing free-fall. Any experiments you could do in either of those cases wouldn’t be able to tell you what was really happening outside your box. The way a ball drops is exactly the same in either case, and you would not be able to tell if you were falling in an elevator shaft or drifting in space.
Please login or register to read the rest of this content.
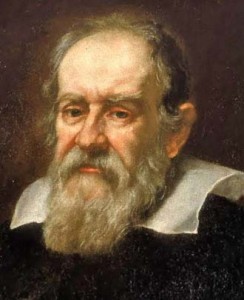
Gravity is the reason behind books being dropped and suitcases feeling heavy. It’s also the reason our atmosphere sticks around and oceans staying put on the surface of the earth. Gravity is what pulls it all together, and we’re going to look deeper into what this one-way attractive force is all about.
Please login or register to read the rest of this content.
One of Newton’s biggest contributions was figuring out how to show that gravity was the same force that caused both objects like an apple to fall to the earth at a rate of 9.81 m/s2 AND the moon being accelerated toward the earth but at a different rate of 0.00272 m/s2. If these are both due to the same force of gravity, why are they different numbers then? Why is the acceleration of the moon 1/3600th the acceleration of objects near the surface of the earth? It has to do with the fact that gravity decreases the further you are from an object. The moon is in orbit about 60 times further from the earth’s center than an object on the surface of the earth, which indicates that gravity is proportional to the inverse of the square of the distance (also called the inverse square law). So the force of gravity acts between any two objects and is inversely proportional to the square of the distance between the two centers. The further apart the objects are, the less they force of gravity is between the two of them. If you separate the objects by twice the distance, the gravitational force goes down by a factor of 4.
Please login or register to read the rest of this content.
All objects are attracted to each other with a gravitational force. You need objects the size of planets in order to detect this force, but everything, everywhere has a gravitational field and force associated with it. If you have mass, you have a gravitational attractive force. Newton’s Universal Law of Gravitation is amazing not because he figured out the relationship between mass, distance, and gravitational force (which is pretty incredible in its own right), but the fact that it’s universal, meaning that this applies to every object, everywhere.
Please login or register to read the rest of this content.
Lord Henry Cavendish in 1798 (about a century after Newton) performed experiments with a torsion balance to figure out the value of G. It’s a very small number, so Cavendish had to carefully calibrate his experiment! The reason the number is so small is because we don’t see the effects of gravity until objects are very massive, like a moon or a planet in size.
Please login or register to read the rest of this content.
How do astronomers find planets around distant stars? If you look at a star through binoculars or a telescope, you’ll quickly notice how bright the star is, and how difficult it is to see anything other than the star, especially a small planet that doesn’t generate any light of its own! Astronomers look for a shift, or wobble, of the star as it gets gravitationally “yanked” around by the orbiting planets. By measuring this wobble, astronomers can estimate the size and distance of larger orbiting objects.
Doppler spectroscopy is one way astronomers find planets around distant stars. If you recall the lesson where we created our own solar system in a computer simulation, you remember how the star could be influenced by a smaller planet enough to have a tiny orbit of its own. This tiny orbit is what astronomers are trying to detect with this method.
Materials
- Several bouncy balls of different sizes and weights, soft enough to stab with a toothpick
- Toothpicks
Weight is nothing more than a measure of how much gravity is pulling on you. This is why you can be “weightless” in space. You are still made of stuff, but there’s no gravity to pull on you so you have no weight. The larger a body is, the more gravitational pull (or in other words the larger a gravitational field) it will have.
The Moon has a fairly small gravitational field (if you weighed 100 pounds on Earth, you’d only be 17 pounds on the Moon). The Earth’s field is fairly large and the Sun has a HUGE gravitational field (if you weighed 100 pounds on Earth, you’d weigh 2,500 pounds on the Sun!).
Please login or register to read the rest of this content.
If you could stand on the Sun without being roasted, how much would you weigh? The gravitational pull is different for different objects. Let’s find out which celestial object you’d crack the pavement on, and which your lightweight toes would have to be careful about jumping on in case you leapt off the planet.
Weight is nothing more than a measure of how much gravity is pulling on you. Mass is a measure of how much stuff you’re made out of. Weight can change depending on the gravitational field you are standing in. Mass can only change if you lose an arm.
Materials
- Scale to weigh yourself
- Calculator
- Pencil
First we’re going to assume the earth is like a ball in that it’s a perfect sphere, and also that the density of the earth is even and it depends only how far from the center of the earth you are. Let’s also assume the earth isn’t rotating. Once we have these things in mind, then the magnitude of the force of gravity acting on an object goes like this…
Please login or register to read the rest of this content.
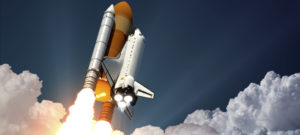
PRE-K & K
Material properties, introduction to forces and motion, plants and animals, and basic principles of earth science.
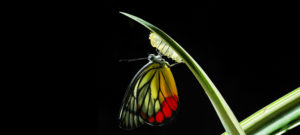
First Grade
States of matter, weather, sound energy, light waves, and experimenting with the scientific method.
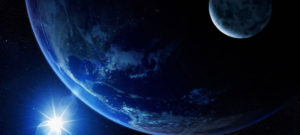
Second Grade
Chemical reactions, polymers, rocks and minerals, genetic traits, plant and animal life cycles, and Earth's resources.
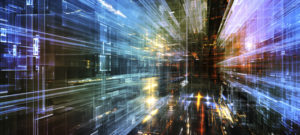
Third Grade
Newton's law of motion, celestial objects, telescopes, measure the climate of the Earth and discover the microscopic world of life.
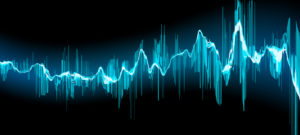
Fourth Grade
Electricity and magnetism, circuits and robotics, rocks and minerals, and the many different forms of energy.
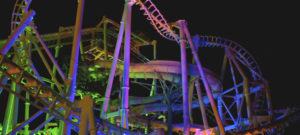
Fifth Grade
Chemical elements and molecules, animal and plant biological functions, heat transfer, weather, planetary and solar astronomy.
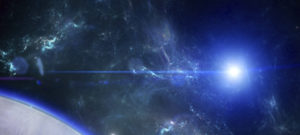
Sixth Grade
Heat transfer, convetion currents, ecosystems, meteorology, simple machines, and alternative energy.
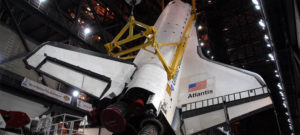
Seventh Grade
Cells, genetics, DNA, kinetic and potential, thermal energy, light and lasers, and biological structures.
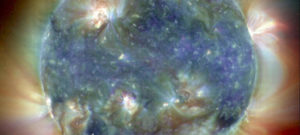
Eighth Grade
Acceleration, forces projectile motion chemical reactions, deep space astronomy, and the periodic table.
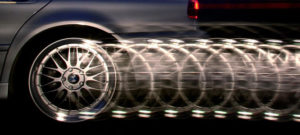
High School (Advanced)
Alternative energy, astrophysics, robotics, chemistry, electronics, physics and more. For high school & advanced 5-8th students.
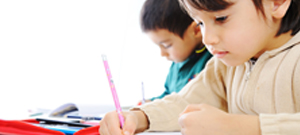
Teaching Resources
Tips and tricks to getting the science education results you want most for your students.
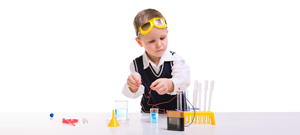
Science Fair Projects
Hovercraft, Light Speed, Fruit Batteries, Crystal Radios, R.O.V Underwater Robots and more!
There are three main differences between assuming the earth is round, uniformly dense, and not rotating as we did before.
First, the crust is not uniform. There are lumps and clumps everywhere that vary the density add up to make small variations in the force of gravity that we can actually measure with objects in free-fall motion. It’s actually how scientists find pockets of oil in the earth. They measure the surface gravity and plot it out, and if there’s a large enough deviation, it means there’s something interesting underground.
This image of the Mors salt dome in Denmark was studied for radioactive waste disposal. It’s a surface gravity survey that measures the acceleration due to gravity that shows something interesting is underground! The dots are the places where gravity was actually measured. You can read more about how gravity is measured from advanced lecture notes here. The unit of measurement for these deviations is called the “milligal” for Galileo, where 1 gal = 1,000 mgal = 1 cm/s3.
Click here to go to next lesson on The Earth is not a Sphere.
[/am4show]
The second problem with our assumption sis that the earth is not a sphere. It’s flattened a bit at the poles and bulges out at the equator. The ring around equator is larger than a ring around the poles by 21 km, which makes the poles closer to the center of the earth than the equator! Free-fall at the poles is slightly more than free-fall at the equator.
But before you book a trip to skydive in Ecuador, Colombia, Brazil, Sao Tome, Gabon, the Republic of the Congo, the Democratic Republic of the Congo, Uganda, Kenya, Somalia, Maldives, Indonesia or Kiribati, let’s talk about the assumption we made… The earth really does rotate. That’s not a surprise. How does this affect the value of g then? The bottom line is that gravity changes with altitude from 9.78 to 9.84 m/s2., mostly due to the earth spinning, but some to the earth not being a perfect sphere.
Please login or register to read the rest of this content.
Circular motion is a little different from straight-line motion in a few different ways. Objects that move in circles are roller coasters in a loop, satellites in orbit, DVDs spinning in a player, kids on a merry go round, solar systems rotating in the galaxy, making a left turn in your car, water through a coiled hose, and so much more.
Please login or register to read the rest of this content.